The 12 hallmarks of ageing refer to key biological processes identified as contributing to the ageing process and age-related diseases. These hallmarks are:
- Genomic Instability
- Telomere Attrition
- Epigenetic Alterations
- Loss of Proteostasis
- Deregulated Nutrient Sensing
- Mitochondrial Dysfunction
- Cellular Senescence
- Stem Cell Exhaustion
- Altered Intercellular Communication
- Inflammaging
- Dysbiosis of the Microbiome
- Impaired Autophagy
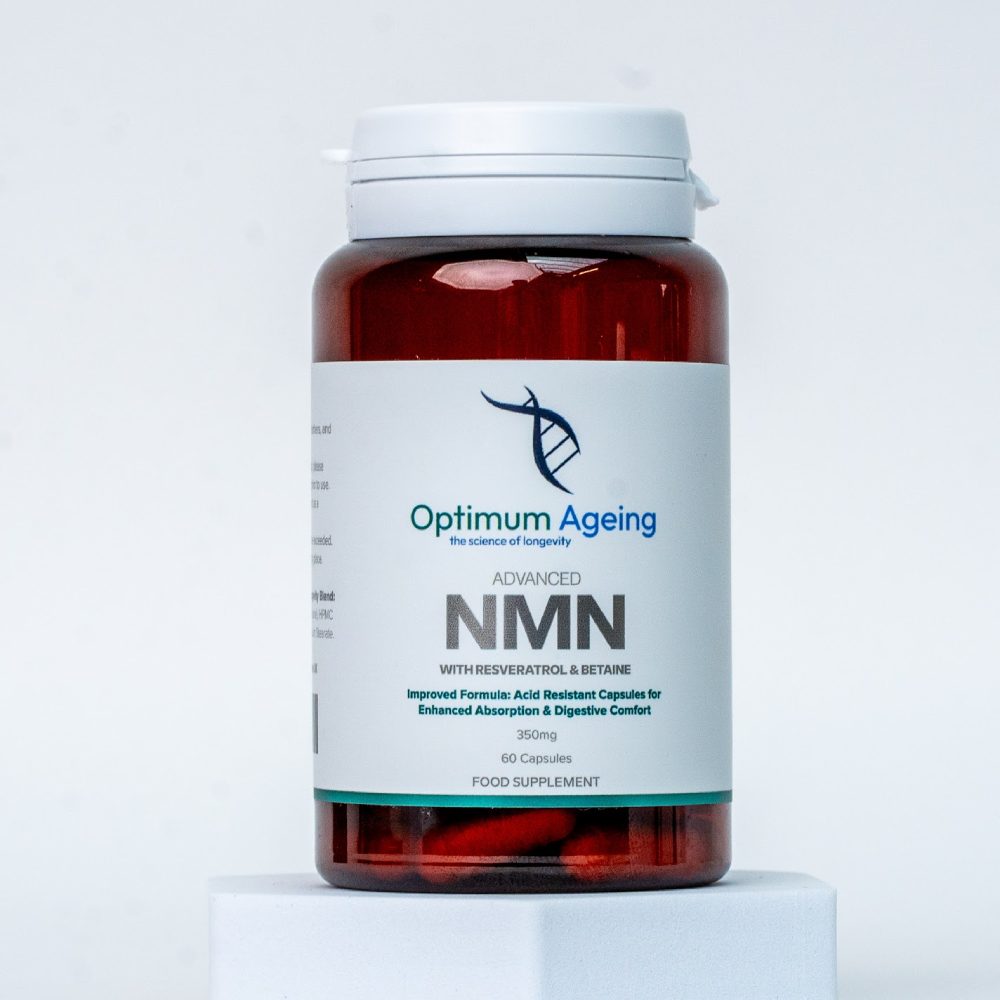
Genomic Instability
To understand genomic instability, first we need to understand:
What’s DNA and how it functions in our body?
Imagine DNA as a vast library of blueprints stored in the factory’s main office. Each blueprint contains detailed instructions for manufacturing different components, from simple tools to complex machinery. These components represent the proteins that perform various functions within the factory.
Now, let’s break down the functions of DNA:
1. Blueprints for Products (Proteins): DNA serves as the repository of blueprints for all the products (proteins) that the factory needs to manufacture. Just like different blueprints specify the materials and assembly instructions for different products, DNA contains the genetic code that specifies the sequence of amino acids needed to build each protein.
2. Assembly Line (Protein Synthesis): Inside the factory, there’s an assembly line where workers (ribosomes) read the blueprints (DNA) and assemble the necessary components (amino acids) to produce the final products (proteins). This process, called protein synthesis, follows the instructions encoded in the DNA to create the precise sequence of amino acids required for each protein.
3. Quality Control (Gene Regulation): The factory’s management closely monitors the production process to ensure that each product is manufactured according to specifications. Similarly, within the body, gene regulation mechanisms oversee the expression of genes (production of proteins) to ensure that they are activated or deactivated at the right time and in the right amount, depending on the body’s needs.
4. Duplication of Blueprints (DNA Replication): To expand the factory or replace damaged blueprints, the management makes copies of the original blueprints.
Similarly, DNA undergoes replication before cell division, ensuring that each new cell receives an identical copy of the genetic information, allowing the factory to continue its operations smoothly.
5. Inheritance (Genetic Transmission): Just as the factory’s success relies on passing down its blueprints and knowledge to future generations of workers, organisms pass down their DNA to their offspring through reproduction. This ensures that the next generation inherits the instructions needed to build and operate their own “factories.”
DNA serves as the fundamental instruction manual that guides the operations of the body, ensuring that the right proteins are produced at the right time and in the right quantities to maintain the factory’s functionality and adaptability to changing conditions.
What’s Genomic Instability?
If imaging our body as a factory, genomic instability can be thought of as a degradation or corruption of the factory’s blueprint (DNA). This concept encompasses various types of damage and errors that accumulate in the genetic material over time, leading to faulty instructions and potentially malfunctioning cellular processes. Here are key aspects of genomic instability:
1. Accumulation of Mutations: Just like errors in the blueprint of a factory can lead to incorrect assembly or malfunction of machines, mutations in DNA can result in the production of dysfunctional proteins or disrupt regulatory processes.
2. DNA Damage: External factors (such as UV radiation, chemicals, or viruses) and internal factors (like reactive oxygen species produced during metabolism) can cause breaks, alterations, or other types of damage to the DNA. This is akin to the blueprint being damaged by external or internal mishaps in the factory.
3. Faulty Repair Mechanisms: Normally, the body has repair mechanisms to fix DNA damage, much like a factory might have a maintenance team to repair or correct errors in the blueprint. When these repair systems fail or become less effective, errors accumulate, leading to genomic instability.
4. Chromosomal Abnormalities: Structural changes in chromosomes, such as deletions, duplications, inversions, or translocations, can disrupt the genetic instructions. In a factory, this would be similar to entire sections of the blueprint being missing, duplicated, or rearranged incorrectly, leading to production errors.
5. Ageing and Disease: As genomic instability increases, cells can become dysfunctional, enter senescence (stop dividing), or undergo apoptosis (programmedcell death). In a factory, repeated blueprint errors can lead to machinery breakdown, inefficient processes, and overall decline in factory productivity. In the body, thiscontributes to ageing and increases the risk of age-related diseases like cancer, where cells proliferate uncontrollably due to corrupted genetic instructions.
In summary, genomic instability in the body’s factory analogy represents the cumulative damage and errors in the DNA blueprint, leading to compromised cellular function, increase drisk of disease, and overall decline in the efficiency and reliability of the body’s systems.
Telomere Attrition
What’s Telomere and its key functions?
Telomeres are repetitive DNA sequences (TTAGGG in humans) located at the ends of linear chromosomes in eukaryotic cells. Their primary function is to protect the integrity of the genome by preventing the loss of genetic information during DNA replication and by shielding the ends of chromosomes from degradation and fusion with neighboring chromosomes.
If think of our body is a factory, telomeres serve as crucial components for maintaining the smooth and efficient operation of the factory (our body) by protecting genetic information, preventing errors, and regulating cell division. Just like how proper maintenance and regulation are essential for a factory’s longevity and productivity, telomeres play a vital role in ensuring the health and function of our cells and tissues:
1. Protective Caps (Genomic Stability): Imagine the chromosomes in our cells as conveyor belts carrying important instructions (genes) for the factory’s operations. At the end of each conveyor belt, there are protective caps called telomeres. These telomeres act like sturdy plastic covers at the end of the conveyor belts, ensuring that the instructions (genes) don’t get damaged or lost during the transportation process.
2. Non-Stick Coating (Prevention of Chromosomal Fusion): Just like how you wouldn’t want the ends of conveyor belts to stick together and cause a mess, telomeres prevent the ends of chromosomes from sticking to each other. They act like a non-stick coating, ensuring that each conveyor belt (chromosome) remains separate and intact, preventing any mix-ups or damage during transportation.
3. Maintenance Crew (Cellular Senescence and Apoptosis): Over time, with each round of cell division, the protective plastic covers (telomeres) at the end of the conveyor belts gradually wear out. When these covers become too short (like running out of plastic cover material), it’s a signal that the conveyor belt (chromosome) is reaching the end of its useful life. At this point, the maintenance crew (cell) stops using that conveyor belt and either repairs it (cellular senescence) or disposes of it (apoptosis), preventing any potential errors or malfunctions in the factory.
4. Mitotic Clock (Regulation of Cell Division): Imagine each cell in the factory has a built-in stopwatch (telomere length) that measures how many times it has divided. As each division occurs, the stopwatch (telomere) ticks down until it reaches a critical point, signaling that the cell has reached its maximum number of divisions. This prevents the cells from dividing indefinitely, ensuring that the factory doesn’t produce too many defective or malfunctioning products (cells).
What’s Telomere Attrition?
Telomere Attrition – Wear and Tear of Protective Caps
In biological terms, every time a cell divides, the telomeres at the ends of chromosomes get slightly shorter. This is because the DNA replication machinery cannot fully replicate the very end of the chromosome, a problem known as the “end replication problem. This is just like each time the conveyor belt is used in the factory, the protective plastic cover at the end wears down a little bit. This gradual shortening of the protective plastic covers (telomeres) is referred to as telomere attrition.
Consequences of Telomere Attrition
Like in the factory, if the protective covers wear out completely, the ends of the conveyor belts are exposed and can become damaged or cause the belts to stick to each other, leading to breakdowns or malfunctions in the factory’s operations. In our body, when telomeres become critically short, the chromosomes are at risk of being recognized as damaged DNA.
This can trigger cellular responses such as:
1. Cellular Senescence: The cell stops dividing and enters a state of permanent growth arrest. Senescent cells can accumulate and contribute to tissue dysfunction and ageing.
2. Apoptosis: The cell undergoes programmed cell death to prevent the propagation of potential genetic errors.
3. Genomic Instability: Exposed chromosome ends can lead to chromosomal fusion and rearrangements, increasing the risk of cancer and other age-related diseases.
As telomeres shorten over time, the increased presence of senescent cells contributes to the decline in tissue function and the appearance of ageing phenotypes. Also, short telomeres are linked to diseases such as cardiovascular disease, certain types of cancer, and conditions related to impaired tissue regeneration.
In summary, telomere attrition represents the natural wear and tear of the protective caps (telomeres) at the ends of chromosomes (conveyor belts) due to repeated cell divisions. This process contributes to cellular ageing and the overall ageing of the organism, as well as to the development of age-related diseases.
Epigenetic Alterations
What’s Epigenetics?
Let’s imagine our body as a highly sophisticated factory. In this factory:
✓ DNA is the master blueprint containing all the instructions needed to manufacture various products (proteins and other molecules).
✓ Genes are specific sections of the blueprint that provide instructions for making particular components or products.
✓ Epigenetics acts as the factory’s management system that controls how, when, and to what extent different parts of the blueprint are read and used without altering the blueprint itself.
Functions of Epigenetics in the Factory
1. DNA Methylation (Instruction Redaction): Imagine the factory managers applying sticky notes (methyl groups) to certain parts of the blueprint. These sticky notes cover up specific instructions (genes) so that the workers (cell machinery) can’t read them.
This prevents certain products from being made when they’re not needed.
2. Histone Modification (Blueprint Accessibility): The blueprint in our factory is wrapped around spools (histones). Sometimes, the spools are tightly wound, making it hard for the workers to access the instructions. Other times, they are loosely wound, making it easier. By adding or removing chemical tags (like adding or removing thread), managers can tighten or loosen the spools, controlling how easily different parts of the blueprint can be read.
3. Non-coding RNA (Instruction Managers): Non-coding RNAs are like middle managers in the factory. They don’t produce products themselves but influence which instructions get followed. Some may intercept and destroy specific instruction sheets (mRNA) before they reach the workers, ensuring that certain products aren’t made.
4. Development and Differentiation (Department Specialization): When the factory was first set up (embryonic development), every department (cell) needed to specialise in producing different products (differentiation).
Epigenetic managers marked specific parts of the blueprint in each department to activate only the instructions needed for that department’s specialised tasks.
5. Gene Regulation (Production Adjustments): Depending on the factory’s needs, the epigenetic managers can quickly adjust production levels. For instance, in response to external conditions (like stress or nutrient availability), they might temporarily increase or decrease the production of certain products by adjusting how the blueprint is read.
6. Memory and Learning (Adaptive Production): For the factory’s R&D department (neurons), keeping track of past projects (memories) is crucial. Epigenetic adjustments allow the department to make long-term changes to the blueprint, ensuring quick access to important instructions based on past experiences, thus improving future project outcomes.
What’s Epigenetic alterations?
Epigenetic alterations can be likened to changes or malfunctions in the factory’s management system. Just as in a factory, where alterations in management practices or systems can lead to production inefficiencies or errors, epigenetic alterations in our body can disrupt normal cellular functions and contribute to various health issues. Here’s how epigenetic alterations might manifest in our factory analogy:
1. DNA Methylation Errors (Sticky Note Mishaps): Imagine if the factory’s management team accidentally applied sticky notes (methyl groups) to the wrong sections of the blueprint. This could result in important instructions being hidden when they shouldn’t be or vice versa, leading to the production of incorrect products or the failure to produce essential ones.
2. Histone Modification Imbalances (Spool Mismanagement): Suppose the factory’s managers start adding or removing thread from the spools (histones) haphazardly.
Some sections of the blueprint might become excessively tightly wound, while others are left overly loose. This could disrupt the proper regulation of gene expression, leading to abnormal production levels or types of products.
3. Non-coding RNA Dysregulation (Middle Manager Mayhem): In a scenario where middle managers (non-coding RNAs) start intercepting and destroying instruction sheets (mRNAs) indiscriminately, essential products might not get made, or unwanted products might accumulate, causing dysfunction in the factory’s operations.
4. Unpredictable Developmental Changes (Departmental Chaos): During the factory’s setup phase (embryonic development), if the epigenetic management team fails to properly specialize different departments (cells), chaos might ensue. Some departments might start producing products they shouldn’t, while others might fail to produce what they’re supposed to, leading to developmental abnormalities.
5. Erratic Gene Regulation (Production Fluctuations): Epigenetic alterations could cause unpredictable fluctuations in production levels across different departments (tissues or organs) of the factory. This could result in inefficient resource allocation, leading to imbalances in product supply and demand.
6. Memory and Learning Deficits (R&D Dysfunction): If the factory’s R&D department (neurons) experiences epigenetic alterations that impair memory formation and learning, it might struggle to retain important project details or learn from past experiences, hindering innovation and problem-solving abilities.
7. Ageing (Wear and Tear Management): Over time, the factory experiences wear and tear. Epigenetic marks can accumulate errors, leading to some instructions being misread or ignored. This can result in reduced efficiency and increased production errors, contributing to the factory’s ageing process
8. Disease Development (Management Breakdown): Ultimately, severe epigenetic alterations could lead to catastrophic breakdowns in the factory’s management system, resulting in the uncontrolled production of defective or harmful products. This could pave the way for the development of various diseases, such as cancer or neurodegenerative disorders.
In summary, epigenetic alterations in our body’s “factory” can disrupt normal cellular functions, leading to a range of health issues and disease states. Understanding and potentially correcting these epigenetic abnormalities is a key focus of research in fields like epigenetics and personalized medicine.
Loss of Proteostasis
Understand what’s Protein and Proteostasis?
1. Protein (Product):
✓ In the factory of our body, proteins are like the products manufactured on the assembly line. They are essential molecules that perform a wide range of functions, acting as enzymes, structural components, signaling messengers, and more.
✓ Just like products in a factory, proteins are made up of smaller components, in this case, amino acids. These amino acids are assembled into chains and then folded into specific three-dimensional shapes, which determine the protein’s function.
✓ Proteins are involved in nearly every aspect of cellular function, from metabolism to DNA replication to cell signaling.
2. Proteostasis (Quality Control):
✓ In any factory, maintaining the quality of the products is crucial to ensure the smooth operation of the assembly line and the satisfaction of customers.
Similarly, in our body factory, proteostasis refers to the quality control mechanisms that ensure proteins are properly synthesised, folded, and degraded.
✓ Proteostasis is like the quality control system in a factory, where various checks and balances are in place to ensure that products meet certain standards before they are released.
✓ Within our cells, proteostasis involves processes such as protein folding, quality control mechanisms that detect and repair misfolded proteins, and pathways for protein degradation.
✓ Just as a malfunction in the quality control system of a factory can lead to defects in products, disruptions in proteostasis can result in the accumulation of misfolded or damaged proteins, which can be harmful to cells and contribute to various diseases, including neurodegenerative disorders and cancer.
By maintaining proteostasis, our body ensures that the proteins produced are of high quality and function properly, ultimately contributing to the overall health and function of the organism, much like how a well-managed factory produces high-quality products and operates efficiently.
Loss of Proteostasis (Breakdown of Quality Control)
In a factory, if the quality control system breaks down, several negative outcomes can occur, impacting the overall efficiency and productivity of the factory. Similarly, in our body, the loss of proteostasis leads to a series of detrimental effects on cellular health and function:
1. Accumulation of Defective Products (Proteins): In a factory, without effective quality control, defective products begin to accumulate on the assembly line. These defective products can clutter the workspace and may even get shipped out, leading to dissatisfied customers and potential recalls. Likewise, in the body, the accumulation of misfolded or damaged proteins occurs. These proteins can form aggregates that disrupt cellular functions. This is particularly problematic in neurons, where protein aggregates are linked to neurodegenerative diseases like Alzheimer’s, Parkinson’s, and Huntington’s disease.
2. Increased Production Costs (Energy and Resource Drain): If defective products aren’t caught early, more resources are wasted on materials and labor to produce products that ultimately can’t be sold or used. In our body, the loss of proteostasis can cause cells expend more energy and resources trying to manage and degrade misfolded proteins. This energy drain can compromise other vital cellular processes, leading to cellular dysfunction and increased vulnerability to stress.
3. Production Line (Cellular Processes) Disruptions: A breakdown in quality control can cause disruptions along the assembly line, slowing down production and causing bottlenecks. Similarly, misfolded proteins can interfere with normal cellular processes. For example, they may clog up the proteasome or autophagy pathways, which are responsible for degrading and recycling cellular waste. This can lead to a toxic cellular environment and increased oxidative stress.
4. Employee Burnout and Turnover (Cellular Stress and Death): If employees are constantly dealing with defective products and production issues, morale can drop, leading to burnout and higher turnover rates. In the body, cellular stress from the accumulation of misfolded proteins can trigger cellular senescence or apoptosis (programmed cell death). This results in the loss of functional cells and contributes to tissue degeneration and ageing.
5. Product Quality (Cellular Function) Decline: The overall quality of products declines if the factory can’t maintain strict quality control, damaging the factory’s reputation and profitability. In our body, the overall functionality of cells declines, affecting tissue health and leading to the progression of age-related diseases. This decline in cellular health is a hallmark of ageing and can manifest in various ways, such as impaired cognitive function, weakened muscles, and decreased regenerative capacity.
In summary, the loss of proteostasis in the body factory disrupts cellular quality control mechanisms, leading to the accumulation of defective proteins, increased cellular stress, and overall decline in cellular and tissue function.
This contributes significantly to the ageing process and the development of age-related diseases.
Deregulated Nutrient Sensing
What’s Nutrient Sensing and How Does It Work?
Imagine your body is a large, complex factory that produces various products (like energy, proteins, and other vital molecules) to keep everything running smoothly. To ensure optimal production, the factory must constantly monitor its supplies and adjust its operations based on the availability of raw materials (nutrients). Nutrient sensing is akin to the factory’s control system that manages this balance. Here’s how it works:
1. Supply Chain Monitoring (Insulin/IGF-1 Signaling Pathway):
✓ Insulin and IGF-1 are like the factory’s sensors that detect incoming shipments of glucose (a key raw material) and other nutrients.
✓ When glucose levels are high, these sensors send signals to increase production activities, ensuring that the factory cells take up glucose and use it
for energy and growth.
2. Main Control Room (mTOR Pathway):
✓ The mTOR Complex 1 (mTORC1) is the main control unit that oversees the production lines for proteins, lipids, and other essential components.
✓ When it detects a plentiful supply of amino acids (building blocks for proteins), it ramps up production and halts the recycling process (autophagy), focusing on growth and synthesis.
3. Energy Department (AMPK Pathway):
✓ The AMP-Activated Protein Kinase (AMPK) is like the energy management department of the factory.
✓ When energy supplies are low (high AMP/ATP ratio), AMPK kicks in to conserve energy, initiating energy-saving processes and halting energy-
consuming ones. It also activates recycling efforts to generate more energy.
4. Efficiency Experts (Sirtuins):
✓ Sirtuins, particularly SIRT1, act as efficiency experts who ensure the factory operates at peak performance, especially during lean times.
✓ They respond to changes in energy levels, optimizing processes to enhance productivity and stress resistance, promoting long-term sustainability of the factory operations.
5. Quality Control (Nutrient Sensing via Amino Acid Sensors):
✓ Sensors like Gcn2 and other components of the mTORC1 pathway are like the quality control team that monitors the availability of specific amino acids.
✓ If there is a shortage, they slow down production to prevent wastage and activate maintenance and stress response mechanisms to preserve factory integrity.
In the context of ageing, these nutrient sensing pathways can become deregulated, akin to a factory’s control systems malfunctioning. Over time, this can lead to inefficiencies, overproduction in some areas, and underproduction in others, ultimately contributing to the factory’s (body’s) decline.
Understanding these pathways allows scientists to explore interventions that might recalibrate the factory’s control systems, improving efficiency, and potentially extending its operational lifespan.
The Consequences of Deregulated Nutrient Sensing
Imagine the nutrient sensing pathways in your body as the control systems of a factory. When these systems become deregulated, it’s like the factory’s control room starts malfunctioning, leading to a cascade of issues that impact the factory’s efficiency and longevity.
1. Overactive Supply Chain Monitoring (Insulin/IGF-1 Signaling Pathway):
✓ When the insulin/IGF-1 signaling pathway is chronically overactive, it’s as if the factory’s sensors constantly report an excess of raw materials, even when there isn’t one.
✓ Consequence: The factory continues to operate at full capacity, overproducing and consuming resources unsustainably. This leads to increased wear and tear on machinery (cells), heightened risk of breakdowns (diseases like cancer), and premature ageing.
2. Faulty Main Control Room (mTOR Pathway):
✓ If the mTOR pathway is persistently active, it’s like the main control unit continuously prioritizes growth and new production, ignoring the need for maintenance and recycling.
✓ Consequence: The factory accumulates waste and damaged parts because recycling processes (autophagy) are suppressed. Over time, this leads to
clogged machinery and inefficient operations, contributing to cellular damage and age-related diseases.
3. Energy Department Mismanagement (AMPK Pathway):
✓ When AMPK is underactive, it’s as if the energy management department fails to recognize low energy supplies and doesn’t activate energy-saving measures.
✓ Consequence: The factory operates wastefully during energy shortages, depleting its reserves and leading to energy crises. This can result in decreased cellular function, increased oxidative stress, and a higher likelihood of metabolic disorders.
4. Ineffective Efficiency Experts (Sirtuins):
✓ If sirtuins are not functioning properly, the factory’s efficiency experts fail to optimise operations and maintain long-term sustainability.
✓ Consequence: The factory becomes less resilient to stress and less efficient in resource usage, leading to reduced productivity and accelerated ageing.
5. Compromised Quality Control (Amino Acid Sensors):
✓ When amino acid sensors like Gcn2 and mTORC1 are deregulated, the quality control team cannot accurately detect and respond to shortages or excesses of specific materials.
✓ Consequence: Production processes are either stalled unnecessarily or continued wastefully, leading to imbalanced production, accumulation of
defective products, and increased cellular stress.
Overall, deregulated nutrient sensing results in a factory that operates inefficiently, wastes resources, accumulates damage, and becomes increasingly prone to breakdowns. In biological terms, this manifests as accelerated ageing, a higher incidence of age-related diseases (like cancer, diabetes, and neurodegenerative disorders), and a decline in overall physiological function.
Mitochondrial Dysfunction
What’s the Role of Mitochondria in our body?
Imagine our body as a factory, with each cell being a separate workshop. In this factory, the mitochondria are the power plants that provide the energy needed to keep all the machinery running smoothly. Here’s how they function in this factory analogy:
1. Power Generation (ATP Production): The mitochondria are the power plants of the cell. They generate electricity (ATP) through a process called oxidative phosphorylation, ensuring that the entire factory has a constant supply of energy to operate all its machines and processes.
2. Metabolic Control Center: These power plants also serve as the control centers for various production lines. They oversee important metabolic pathways, like the Krebs cycle, which breaks down raw materials (nutrients) to produce energy and essential building blocks for the factory’s operations.
3. Calcium Regulation (Calcium Homeostasis): The mitochondria manage the storage and release of essential materials like calcium, much like a warehouse that controls the supply of critical components needed for various factory processes, such as signaling between different departments (cell functions).
4. Quality Control and Safety (Apoptosis): In case of major malfunctions or damages, the mitochondria act as a safety control center. They can initiate a shutdown process (apoptosis) to prevent further damage to the factory, ensuring that defective parts do not compromise the overall operation.
5. Waste Management (ROS Production): During energy production, mitochondria also handle waste byproducts (reactive oxygen species). They ensure that waste levels are kept in check, but if the waste becomes excessive, it can cause damage to the factory’s machinery, highlighting the need for proper waste management systems.
6. Heat Production (Thermogenesis): In specialised areas of the factory, mitochondria can switch to producing heat instead of electricity. This process, similar to turning on a heating system, helps maintain optimal operating temperatures in specific parts of the factory.
7. Material Synthesis (Biosynthesis of Macromolecules): Mitochondria are also involved in producing essential materials and components, such as certain lipids and hormones, much like a dedicated section of the factory that manufactures specialised products needed for the factory’s functions.
8. Redox Balance (Regulation of Redox Status): They help maintain the factory’s internal environment by regulating the balance of reducing agents (redox status), which are crucial for keeping all processes running efficiently and preventing machinery from rusting or corroding (oxidative damage).
In summary, in our body-as-a-factory analogy, mitochondria are versatile power plants that not only provide energy but also manage key aspects of production, safety, waste management, and environmental control, ensuring the factory runs smoothly and efficiently.
The Consequences of Mitochondrial Dysfunction
In our body-as-a-factory analogy, if the mitochondria, which serve as the power plants and control centers of the cell, experience dysfunction, it can have significant consequences for the overall operation of the factory (i.e., the body). Here are some potential consequences of mitochondrial dysfunction based on the roles described earlier:
1. Energy Shortages: Mitochondrial dysfunction can lead to decreased ATP production, akin to a power outage in the factory. This energy shortage can result in fatigue, weakness, and impaired function of tissues and organs throughout the body.
2. Metabolic Imbalances: Dysfunction in mitochondrial metabolic pathways can disrupt the production of essential molecules and energy substrates needed for various cellular processes, potentially leading to metabolic disorders and impaired tissue function.
3. Calcium Dysregulation: Alterations in calcium handling by dysfunctional mitochondria can disrupt cellular signaling pathways, leading to disturbances in processes such as muscle contraction, neurotransmitter release, and enzyme activity.
4. Impaired Quality Control: Dysfunctional mitochondria may fail to initiate apoptosis properly, leading to the accumulation of damaged cells and potentially contributing to the development of cancer or other diseases.
5. Increased Oxidative Stress: Dysfunction in mitochondria can lead to excessive production of reactive oxygen species (ROS), overwhelming the cell’s antioxidant defenses and causing oxidative damage to proteins, lipids, and DNA.
6. Reduced Thermogenesis: Dysfunction in mitochondria’s ability to produce heat can impair the body’s ability to regulate temperature, potentially leading to problems with thermoregulation and susceptibility to cold exposure.
7. Decreased Biosynthesis: Mitochondrial dysfunction can disrupt the synthesis of essential molecules and hormones, potentially leading to deficiencies and dysfunction in various systems of the body.
8. Redox Imbalance: Dysfunction in mitochondrial redox balance can disrupt cellular homeostasis and contribute to oxidative damage, inflammation, and cellular dysfunction, ultimately leading to the development of various diseases.
Overall, mitochondrial dysfunction can have far-reaching consequences for cellular and tissue function, contributing to a wide range of health issues, including metabolic disorders, neurodegenerative diseases, cardiovascular diseases, and ageing-related conditions.
Cellular Senescence
What’s cells’ functions?
Cells are like the workers in our body factory. They are responsible for various tasks and operations within the facility. Here’s an overview of cells’ functions in the body, framed within the context of a factory:
1. Structural Support (Construction Crew): Cells act as the construction crew, building and maintaining the infrastructure of the factory. They form the structural framework of tissues and organs, providing support, stability, and shape.
2. Nutrient Transport and Exchange (Logistics Department): Cells function like the logistics department, managing the transportation and distribution of raw materials (nutrients) and finished products (metabolites) throughout the factory. They regulate the intake, transport, and exchange of substances across cell membranes to ensure that all areas of the factory receive the necessary supplies.
3. Metabolism (Production Line): Cells operate like the production line, where various metabolic processes take place. They manufacture essential molecules such as proteins, carbohydrates, and lipids, as well as generate energy through processes like cellular respiration. Specialised cellular structures, such as mitochondria and the endoplasmic reticulum, serve as the factory machinery for these metabolic activities.
4. Cellular Communication (Communication Network): Cells function as the communication network of the factory, transmitting signals and messages to coordinate activities and respond to changes in the environment. Cell signaling pathways allow cells to communicate with each other, regulating processes such as growth, development, and response to stimuli.
5. Defense and Immunity (Security and Maintenance Crew): Certain cells, akin to the security and maintenance crew, are responsible for defending the factory against intruders (pathogens) and maintaining a clean and orderly environment. Cells of the immune system identify and neutralise harmful agents, as well as remove damaged or abnormal cells to ensure the smooth operation of the factory.
6. Reproduction and Growth (Expansion Team): Cells play a role similar to the expansion team, responsible for reproduction and growth within the factory. They produce offspring (via cell division) and contribute to the growth and development of tissues and organs, ensuring that the factory can adapt and expand as needed.
7. Sensory Reception (Sensors): Specialized cells, acting as sensors, detect and transmit sensory information from the external environment to the central control center (the brain and nervous system). They allow the factory to respond to stimuli such as light, sound, touch, taste, and smell, facilitating appropriate reactions and adjustments.
8. Homeostasis (Quality Control): Cells function as the quality control department, monitoring and maintaining internal stability and balance within the factory. They regulate factors such as temperature, pH, osmotic pressure, and ion concentrations to ensure optimal conditions for cellular functions and overall factory performance.
In essence, cells are the dynamic workforce of the body, carrying out a multitude of specialised tasks and functions to ensure the smooth operation and maintenance of the “factory” that is the human body.
What does cellular senescence lead to?
Imagine cellular senescence as a situation where some of the factory workers reach retirement age but continue to linger around the factory without actively contributing to production or maintenance tasks. Here’s how this scenario might unfold:
1. Reduced Efficiency (Decreased Workforce): As more cells enter a senescent state, akin to workers retiring, the overall workforce of the factory diminishes. This reduction in the number of active workers can lead to decreased efficiency in carrying out essential tasks, such as production, maintenance, and response to environmental challenges.
2. Increased Waste and Dysfunction (Inactive Workers): Senescent cells, like inactive workers, may accumulate within the factory environment without actively participating in cellular functions. These cells can become metabolically active, secreting pro-inflammatory molecules and other harmful substances, akin to retired workers causing disruptions within the factory. This can lead to increased cellular waste and dysfunction, contributing to tissue inflammation and damage.
3. Compromised Repair and Regeneration (Limited Maintenance Crew): Senescent cells, similar to retired workers, are no longer capable of fulfilling their roles in tissue repair and regeneration. This can result in compromised maintenance of tissues and organs, leading to a gradual decline in overall factory functionality and resilience to damage.
4. Inflammatory Environment (Labor Disputes): The presence of senescent cells within the factory can create an inflammatory environment, analogous to labor disputes or conflicts among workers. Senescent cells secrete pro-inflammatory molecules, attracting immune cells and exacerbating tissue inflammation. This chronic low-grade inflammation can contribute to the development and progression of various age-related diseases, such as arthritis, cardiovascular disease, and neurodegenerative disorders.
5. Impaired Adaptation to Stress (Limited Flexibility): A workforce containing a significant proportion of senescent cells may have limited capacity to adapt to environmental stressors or challenges, similar to a factory with a reduced and inefficient workforce. This impaired ability to respond to stressors can compromise the factory’s resilience and ability to maintain homeostasis in changing conditions.
In summary, cellular senescence can have significant consequences within the “factory” of the human body, leading to reduced efficiency, increased dysfunction, impaired tissue repair, chronic inflammation, and diminished resilience to stressors. Understanding and addressing cellular senescence are crucial for promoting healthy ageing and mitigating the risk of age-related diseases.
Stem Cell Exhaustion
Stem Cells – Workers with special skills
Stem cells are unique cells with the remarkable ability to develop into many different cell types in the body during early life and growth. If we think of our body as a factory, stem cells are like the workers with the specialized skills. Here’s how stem cells function:
1. Tissue Repair and Regeneration (Maintenance Workers): Stem cells act as maintenance workers in the factory, constantly repairing and replenishing damaged or worn-out parts of the machinery. Just like these workers replace faulty components to keep the factory running smoothly, stem cells replace damaged or ageing cells in our tissues and organs to maintain their function and integrity.
2. Development and Growth (Builders and Architects): During the early stages of life, stem cells serve as the builders and architects of the factory. They lay down the blueprint for how the factory should be constructed and oversee the construction process, ensuring that each part is built correctly and in the right place. Similarly, stem cells in the embryo differentiate into various cell types and structures, guiding the development and growth of tissues and organs.
3. Homeostasis (Janitors): Stem cells act as janitors in the factory, responsible for keeping everything clean and in working order. Just as janitors sweep away debris and maintain cleanliness to ensure a healthy work environment, stem cells continuously replenish the supply of specialized cells in tissues with a high turnover rate, such as the skin and the lining of the intestine, to maintain tissue homeostasis and functionality.
4. Immune System Regulation (Security Guards): Certain types of stem cells, such as mesenchymal stem cells (MSCs), function as security guards in the factory, keeping a watchful eye on intruders and maintaining order. Like security guards who regulate access and respond to threats, MSCs modulate the immune response and help regulate inflammation, playing a critical role in immune system regulation and tissue repair.
5. Drug Discovery and Disease Modeling (Researchers and Testers): Stem cells serve as researchers and testers in the factory’s laboratory, exploring new ideas and testing potential solutions to improve efficiency and productivity. Similarly, stem cells are used in research to study disease mechanisms and screen potential drugs or therapies.
Induced pluripotent stem cells (iPSCs) can be differentiated into various cell types affected by diseases, allowing researchers to model disease processes and test potential treatments in a controlled environment.
In summary, stem cells are like versatile workers in the factory of our body, performing a variety of essential functions to ensure the proper functioning, repair, and maintenance of tissues and organs throughout life.
The Consequences of Stem Cell Exhaustion
Stem cell exhaustion can be likened to a shortage of skilled workers in the factory. Here’s how stem cell exhaustion can affect people’s health:
1. Decreased Tissue Repair and Regeneration: With fewer maintenance workers available, the factory struggles to keep up with repairs and maintenance. Similarly, stem cell exhaustion leads to a decreased capacity for tissue repair and regeneration in the body. This can result in slower healing times, reduced tissue function, and an increased susceptibility to injury and disease.
2. Impaired Development and Growth: Without enough builders and architects, the factory’s expansion and growth plans are put on hold. Similarly, stem cell exhaustion can impair developmental processes and growth in the body, leading to developmental abnormalities or growth disorders.
3. Disrupted Homeostasis: With fewer janitors to maintain cleanliness and order, the factory becomes less efficient and more prone to breakdowns. Similarly, stem cell exhaustion disrupts tissue homeostasis, leading to a decline in overall tissue function and an increased risk of age-related diseases.
4. Compromised Immune Regulation: When security guards are understaffed, the factory becomes vulnerable to security breaches and chaos. Similarly, stem cell exhaustion can compromise immune system regulation, leading to an increased risk of infections, autoimmune diseases, and impaired tissue repair.
5. Limited Capacity for Research and Testing: With fewer researchers and testers available, the factory struggles to innovate and find solutions to emerging challenges.
Similarly, stem cell exhaustion limits the capacity for research and testing in the body, making it harder to understand disease mechanisms and develop new treatments.
Overall, stem cell exhaustion has widespread consequences for the body, affecting tissue repair, development, homeostasis, immune regulation, and research capacity. Maintaining healthy stem cell populations is essential for promoting tissue health, longevity, and overall well-being.
Altered Intercellular Communication
If we think of the body as a factory with cells as workers, intercellular communication serves as the communication network that ensures the smooth operation and coordination of different departments within the factory. Here’s how it parallels:
1. Coordination of Cellular Activities: Just like different departments in a factory need to coordinate their activities to achieve production goals, cells communicate with each other to coordinate their functions and ensure that the body’s processes run smoothly.
2. Regulation of Growth and Development: In a factory, managers provide instructions to workers on what tasks to perform and how to do them efficiently.
Similarly, signaling molecules provide instructions to cells, guiding their growth, proliferation, and differentiation during development and tissue repair.
3. Immune Response: In a factory facing an external threat or malfunction, an alarm system alerts security and maintenance teams to respond appropriately.
Similarly, intercellular communication in the body alerts immune cells to respond to pathogens and coordinate the defense against infections.
4. Maintenance of Tissue Homeostasis: Just as maintenance crews ensure that machinery operates smoothly and facilities remain clean, cells communicate to maintain the balance and integrity of tissues and organs, regulating processes such as nutrient uptake, waste removal, and oxygen supply.
5. Adaptation to Environmental Changes: Factories must adapt to changes in market demands, supply shortages, or equipment failures. Similarly, cells communicate to respond to changes in the external environment or internal conditions, such as stress, injury, or fluctuations in nutrient availability.
6. Integration of Physiological Responses: In a factory, different departments must work together to achieve overall production goals. Similarly, intercellular communication integrates physiological responses between different tissues and organ systems, ensuring that the body functions as a coordinated whole.
The Effect of Altered Intercellular Communication
Altered intercellular communication could be akin to disruptions or malfunctions in the communication network within the factory. Here are some consequences of altered intercellular communication:
1. Coordination Breakdown: Just as disruptions in communication can lead to chaos and inefficiency in a factory, altered intercellular communication can disrupt the coordination of cellular activities in the body. This can result in dysregulated processes, impaired tissue function, and ultimately, disease.
2. Miscommunication in Growth and Development: Similar to how unclear instructions or miscommunication can lead to errors in production or inefficient use of resources in a factory, altered intercellular communication can lead to errors in cell growth, differentiation, and tissue development. This may result in developmental disorders or abnormal tissue growth, such as tumors.
3. Immune Dysfunction: In a factory, a breakdown in communication between security teams and other departments could compromise the response to threats or emergencies. Similarly, altered intercellular communication can impair the immune response, leaving the body vulnerable to infections, autoimmune disorders, or chronic inflammation.
4. Tissue Dysfunction and Homeostatic Imbalance: Disruptions in communication within a factory can lead to machinery breakdowns or inefficiencies in production.
Likewise, altered intercellular communication can disrupt the balance and integrity of tissues and organs, leading to impaired function, compromised tissue repair, and susceptibility to diseases such as metabolic disorders or organ failure.
5. Inability to Adapt to Environmental Changes: Just as a factory that fails to adapt to changes in market demands or supply shortages may struggle to remain operational, altered intercellular communication can impair the body’s ability to adapt to changes in the external environment or internal conditions.
This may result in increased susceptibility to stress, injury, or disease.
6. Disrupted Integration of Physiological Responses: In a factory, disruptions in communication between different departments can hinder the overall production process. Similarly, altered intercellular communication can disrupt the integration of physiological responses between different tissues and organ systems, leading to systemic imbalances and dysfunction.
Altered intercellular communication can have profound effects on the ageing process by disrupting essential cellular and physiological processes, accelerating cellular senescence, impairing tissue repair and regeneration, and promoting inflammation and metabolic dysfunction. Addressing these communication deficits may offer promising avenues for interventions to slow down the ageing process and reduce the risk of age-related diseases.
Chronic Inflammation
Chronic inflammation is a persistent, low-grade inflammatory response that can occur in various tissues and organs throughout the body. Unlike acute inflammation, which is a short-term and beneficial response to tissue injury or infection, chronic inflammation persists over a longer period and can contribute to the development and progression of many age-related diseases.
Chronic inflammation can be triggered by a variety of factors, including:
✓ Persistent Infections: Certain infections, such as hepatitis C virus (HCV) or Helicobacter pylori (H. pylori) infection, can lead to chronic inflammation if not properly controlled by the immune system.
✓ Autoimmune Disorders: Conditions where the immune system mistakenly attacks the body’s own tissues, such as rheumatoid arthritis, systemic lupus erythematosus (SLE), or inflammatory bowel disease (IBD), can result in chronic inflammation.
✓ Obesity: Adipose tissue in obese individuals can release pro-inflammatory cytokines and adipokines, contributing to a chronic inflammatory state.
✓ Environmental Factors: Exposure to environmental pollutants, toxins, or irritants can trigger chronic inflammation in the lungs (e.g., from cigarette smoke) or other tissues.
✓ Unhealthy Lifestyle Factors: Poor diet, lack of exercise, chronic stress, and inadequate sleep can all contribute to chronic inflammation.
If we think of our body as a factory, chronic inflammation can be likened to a malfunction in the production line that causes ongoing damage and disruption to the factory’s operations.
Imagine that in one corner of the factory, there’s a team of workers (immune cells) who are responsible for dealing with any issues that arise, such as repairing machinery (tissues) or fighting off intruders like harmful bacteria or viruses. Normally, these workers are efficient and effective, swiftly resolving problems and maintaining order. However, in the case of chronic inflammation, there’s a constant alarm sounding in this corner of the factory.
It’s as if there’s a never-ending emergency, and the workers are constantly on high alert, releasing signals (pro-inflammatory molecules) to call for reinforcements and activate repair processes.
Initially, this heightened response might be necessary to address a specific issue, like a persistent infection or injury. But imagine if the alarm never shuts off. The workers become overworked and stressed, and their efforts to fix the problem become less effective over time.
As a result, the machinery starts to wear down, and the factory becomes less efficient.
Chronic inflammation can lead to damage in various parts of the factory (organs and tissues), impairing their function and increasing the risk of breakdowns (the development of various diseases, including cardiovascular disease, diabetes, neurodegenerative diseases, and certain types of cancer).
Addressing chronic inflammation is important for overall health and well-being. It is like finding the source of the persistent alarm and restoring calm to that corner of the factory. It involves identifying and addressing the underlying causes of inflammation, whether it’s an ongoing infection, autoimmune condition, unhealthy lifestyle habits, or other factors. By quieting the alarm and restoring balance to the factory, the workers can go back to their normal duties, and the overall operation can run more smoothly, promoting better health and well-being.
Dysbiosis
Dysbiosis refers to an imbalance or maladaptation in the composition of the microbiota, the community of microorganisms (bacteria, viruses, fungi, and other microbes) living in and on our bodies, particularly in the gut. If imagine our body as a factory, the community of microorganisms are like a specialized team of workers who manage key processes in this factory. Dysbiosis can be compared to these workers going on strike or not doing their jobs properly. This disruption in the normal microbial ecosystem can lead to negative health outcomes.
Causes of Dysbiosis
✓ Antibiotic Use: Overuse or inappropriate use of antibiotics can kill beneficial bacteria along with harmful ones.
✓ Diet: Poor dietary choices, such as high-fat, high-sugar, or low-fiber diets, can disrupt the balance of gut microbiota.
✓ Infections: Gut infections can lead to imbalances.
✓ Chronic Stress: Stress can affect gut permeability and microbial balance.
✓ Environmental Toxins: Exposure to pollutants and chemicals can impact the microbiome.
✓ Lack of Physical Activity: Sedentary lifestyle can influence gut health.
How Dysbiosis Relates to Ageing
1. Chronic Inflammation (Inflammaging) Mechanism: Dysbiosis can lead to increased intestinal permeability, often referred to as “leaky gut.” This allows bacteria and their toxins (like lipopolysaccharides, LPS) to enter the bloodstream, triggering a systemic inflammatory response.
✓ Impact: Chronic low-grade inflammation is a hallmark of ageing (inflammaging) and contributes to many age-related conditions, including cardiovascular disease, diabetes, and neurodegenerative disorders .
2. Immune System Dysregulation
✓ Mechanism: The gut microbiota plays a critical role in training and regulating the immune system. Dysbiosis can impair this regulation, leading to an overactive immune response or immune senescence, where the immune system becomes less effective.
✓ Impact: Older adults often experience a decline in immune function
(immunosenescence), increasing susceptibility to infections and reducing vaccine efficacy. Dysbiosis can exacerbate this process .
3. Metabolic Changes
✓ Mechanism: Gut bacteria influence metabolism by interacting with dietary
components, producing short-chain fatty acids (SCFAs) like butyrate, and regulating energy balance. Dysbiosis can disrupt these processes, leading to metabolic dysfunction.
✓ Impact: This can contribute to insulin resistance, obesity, and type 2 diabetes, all of which are associated with ageing and age-related decline .
4. Neurodegenerative Diseases and Cognitive Decline
✓ Mechanism: The gut-brain axis involves bidirectional communication between the gut and the brain. Dysbiosis can affect this axis by altering the production of neurotransmitters, modulating inflammation, and affecting the integrity of the blood-brain barrier.
✓ Impact: Dysbiosis has been linked to neurodegenerative diseases such as Alzheimer’sand Parkinson’s, and to cognitive decline, potentially through mechanisms like increased inflammation and altered gut-derived metabolites .
5. Reduced Nutrient Absorption
✓ Mechanism: Gut bacteria are involved in the digestion and absorption of nutrients, including vitamins (like B vitamins and vitamin K) and minerals. Dysbiosis can impair these processes.
✓ Impact: Malabsorption of essential nutrients can contribute to age-related deficiencies, weakened bone health, and overall frailty .
6. Compromised Gut Barrier Function
✓ Mechanism: A healthy gut barrier prevents harmful substances from entering the bloodstream. Dysbiosis can weaken this barrier, allowing endotoxins and other harmful molecules to pass through.
✓ Impact: This can lead to systemic inflammation and is associated with conditions such as atherosclerosis and metabolic syndrome, which are more prevalent in older adults .
7. Altered Microbial Metabolites
✓ Mechanism: Gut bacteria produce various metabolites, including SCFAs, bile acids, and indole derivatives, which can influence host physiology. Dysbiosis alters the production of these metabolites, affecting processes like inflammation, metabolism, and cellular health.
✓ Impact: Changes in microbial metabolites are linked to age-related diseases and decline in organ function.
Dysbiosis and ageing are intricately connected through mechanisms involving inflammation, immune regulation, metabolism, and the gut-brain axis. Addressing dysbiosis through lifestyle, dietary interventions, and possibly medical treatments may offer potential pathways to mitigate age-related decline and enhance healthy ageing.
Compromised Autophagy
Autophagy is a crucial cellular process. In our body-factory, autophagy acts like the recycling and waste disposal system. Its job is to identify and break down damaged or old cellular components (like machinery and equipment in a factory) that are no longer functional or could be harmful if left unchecked.
Just as in a factory, where broken or obsolete machinery needs to be removed to maintain efficiency and safety, autophagy ensures that cellular
components are continually recycled or disposed of properly. This process helps maintain cellular homeostasis, energy balance, and the removal of potentially harmful cellular debris.
Compromised autophagy refers to the decline in the efficiency or function of the autophagy-lysosomal pathway as organisms age.
Impact of Compromised Autophagy:
When autophagy is compromised, this recycling and waste management system becomes less efficient:
✓ Accumulation of Damaged Components: Damaged organelles (like malfunctioning machines) and misfolded proteins (like defective parts) start to accumulate within cells.
✓ Cellular Dysfunction: As these damaged components build up, they can impair normal cellular functions, much like how a factory’s productivity and efficiency decline when there are broken machines lying around.
✓ Increased Risk of Problems: Just as a factory with a faulty waste management system might face safety hazards or reduced production, compromised autophagy increases the risk of cellular dysfunction, inflammation, and diseases.
Interventions and Maintenance
To optimise autophagy and maintain a healthy recycling system in our body-factory:
✓ Proper Maintenance: Ensuring that autophagy pathways are activated and
functioning well through factors like diet (e.g., intermittent fasting) and exercise.
✓ Supporting Cellular Health: Using tools (such as pharmacological agents) that can help stimulate autophagy pathways, much like how a factory might use maintenance routines and upgrades to keep its systems running smoothly.
In conclusion, compromised autophagy is a significant contributor to the ageing process by allowing the accumulation of cellular damage, promoting inflammation, and contributing to the development of age-related diseases like neurodegenerative disorders and cardiovascular diseases. Maintaining optimal autophagy through lifestyle interventions (such as diet and exercise) or therapeutic approaches holds promise for promoting healthy ageing and extending healthspan, thereby improving overall quality of life as we grow older.